By sheer coincidence, you’ve stumbled upon a topic that holds the key to unlocking the secrets of efficient electric generation through Faraday induction.
Curious to know more? Well, get ready to dive into the realm of electromagnetic wonders as we explore the 9 best methods for harnessing this powerful phenomenon.
From manipulating magnetic fields to optimizing coil designs and utilizing efficient wire materials, these techniques promise to revolutionize the way we generate electricity.
But that’s just the beginning. So, hold on tight and prepare for the possibilities that lie ahead.
Key Takeaways
- Increasing the number of turns in a coil intensifies the induced magnetic field, resulting in higher current and greater electric generation.
- The shape of the coil plays a crucial role in inducing current, so choose a shape that maximizes the magnetic field.
- Select a conductive material with high magnetic permeability to enhance the coil’s magnetic field and improve induction performance.
- Copper and aluminum are commonly used wire materials due to their high electrical conductivity, which is crucial for maximizing the efficiency and performance of electrical generation systems.
Magnetic Field Manipulation
How can you manipulate magnetic fields to enhance electric generation using Faraday induction methods?
Magnetic field manipulation is a crucial aspect of electric generation through Faraday induction. By altering the strength or direction of a magnetic field, you can effectively control the induced field and enhance the generation of electric current.
One method of magnetic field manipulation is to change the number of turns in a coil. Increasing the number of turns intensifies the induced magnetic field, resulting in a higher current and greater electric generation. Conversely, decreasing the number of turns weakens the induced field, leading to lower electric generation.
Additionally, varying the speed or strength of the magnetic field can influence the induced electromotive force (EMF). Understanding Faraday’s Law of induction and Lenz’s Law is essential for effective magnetic field manipulation.
Faraday’s Law states that the induced EMF is proportional to the rate of change of magnetic flux through a coil. Therefore, by manipulating the magnetic flux, you can control the induced EMF and enhance electric generation through electromagnetic induction.
Coil Design Optimization
To optimize the design of your coil for efficient induction, you need to consider three key points.
- The shape of the coil plays a crucial role in inducing current, so choose a shape that maximizes the magnetic field.
- Select a conductive material with high magnetic permeability to enhance the coil’s magnetic field and improve induction performance.
- Balancing the trade-off between the number of turns and wire thickness is essential for achieving optimal coil design.
Keep these points in mind to ensure the highest efficiency in your coil design for Faraday induction methods.
Efficient Coil Shape
Efficient coil shape optimization maximizes the number of turns and minimizes resistance, crucial factors that impact the magnetic flux density and efficiency of electromagnetic induction.
The shape of the coil plays a significant role in determining the uniformity of the magnetic field and the induced voltage. Using a cylindrical or solenoidal shape for coils can enhance the magnetic field’s uniformity, leading to increased induction efficiency.
When optimizing the shape of coils, you need to consider factors such as coil length, diameter, and spacing between turns. By maximizing the flux linkage and minimizing energy losses, the design optimization of coils ensures efficient electric generation.
This allows for the effective conversion of magnetic flux into electrical energy, resulting in an electromagnetic force (EMF) that can power various devices.
Magnetic Field Optimization
Coil design optimization plays a critical role in maximizing the efficiency of electromagnetic induction systems by enhancing the strength and uniformity of the induced magnetic field.
Here are four key factors to consider when optimizing the magnetic field in a coil design:
- Number of Turns: The number of turns in the coil directly affects the strength of the induced magnetic field. By optimizing this parameter, you can increase the overall efficiency of the induction system.
- Shape and Size of the Coil: Adjusting the shape and size of the coil can improve the magnetic flux density. This optimization technique helps in enhancing the overall performance of the induction system.
- Core Material Selection: Choosing the appropriate core material for the coil helps in concentrating the magnetic field, leading to reduced energy losses and improved efficiency.
- Resistance and Inductance: Optimizing the coil’s resistance and inductance based on the frequency and current requirements is crucial to achieve the desired induction efficiency.
Conductive Material Selection
When considering the optimization of coil design for electromagnetic induction systems, one crucial aspect to address is the selection of the conductive material.
The choice of material directly affects the efficiency and performance of the coil in converting changing magnetic fields into induced voltage. To assist in your decision-making process, below is a table comparing the properties of different conductive materials commonly used in wire wound coils:
Material | Electrical Conductivity (S/m) | Magnetic Permeability (H/m) | Resistivity (Ω.m) |
---|---|---|---|
Copper | 5.96 × 10^7 | 1.26 × 10^-6 | 1.68 × 10^-8 |
Aluminum | 3.77 × 10^7 | 1.26 × 10^-6 | 2.65 × 10^-8 |
Silver | 6.30 × 10^7 | 1.26 × 10^-6 | 1.59 × 10^-8 |
Iron | 1.00 × 10^6 | 8.85 × 10^-2 | 9.71 × 10^-8 |
These materials have distinct characteristics that influence the coil’s performance. For example, copper exhibits high electrical conductivity, making it an excellent choice for maximizing the induced EMF. On the other hand, iron has a higher magnetic permeability, enhancing the magnetic field strength within the coil.
Consider these properties carefully when selecting the conductive material for your coil design, as it can significantly affect the efficiency and effectiveness of your electromagnetic induction system.
Efficient Wire Materials
When considering efficient wire materials for electric generation systems, you should take several key points into account.
- Optimal wire conductivity is crucial, with copper and aluminum being commonly used due to their high electrical conductivity.
- The suitable wire thickness must be determined to ensure the desired level of electrical resistance and efficiency.
- The chosen wire material should be resistant to corrosion to maintain its performance over time.
These factors play a critical role in maximizing the efficiency and performance of electrical generation systems.
Optimal Wire Conductivity
Efficient wire materials with high electrical conductivity play a crucial role in achieving optimal wire conductivity for electric generation systems. The choice of wire material impacts the efficiency and performance of these systems.
Here are four key points to consider regarding optimal wire conductivity:
- Copper and aluminum are commonly used efficient wire materials due to their high conductivity. They allow for efficient flow of electric current, reducing energy losses.
- Silver is the most conductive metal; however, its high cost makes it less practical for widespread use in electric generation systems.
- Optimal wire conductivity reduces energy losses and enhances the overall efficiency of electric generation. This is achieved by minimizing resistance and maximizing the flow of current.
- The generation of electric current through Faraday induction is based on the principle of a changing magnetic field inducing an Electromotive Force (EMF) in coils of wire. This EMF causes current to flow, following the Lenz law.
Suitable Wire Thickness
To achieve optimal efficiency in conducting induced current, it’s essential to select wire materials with a suitable thickness. The thickness of the wire affects its resistance, which in turn impacts the efficiency of current flow.
According to Faraday’s Law, when a magnetic field changes in strength or direction, it induces an electric current in a nearby conductor. The magnetic field around a moving magnet creates a motional electromotive force (emf), resulting in a current going through the wire.
To minimize resistance and maximize efficiency, use wires with a larger cross-sectional area. Copper and aluminum are commonly used efficient wire materials due to their high conductivity. By choosing the right wire gauge (AWG) based on the application’s current and voltage requirements, you can optimize efficiency.
Additionally, consider the environmental conditions and mechanical strength requirements when selecting wire materials for durability and long-term performance.
Resistant to Corrosion
Wire materials that are resistant to corrosion, such as copper or aluminum, are crucial for ensuring longevity and reliability in electromagnetic induction systems. The choice of wire materials in these systems is important as they need to withstand the environmental conditions and the constant exposure to induced currents.
Here are four key points to consider:
- Copper, with its excellent electrical conductivity and corrosion resistance, is widely used in electromagnetic induction systems. It allows for efficient current flow and minimizes energy loss.
- Aluminum, although less conductive than copper, is also used as a corrosion-resistant alternative. It offers a cost-effective solution while still maintaining acceptable conductivity levels.
- Protective coatings like stainless steel or specialized alloys can enhance the corrosion resistance of wire materials. These coatings act as a barrier, preventing corrosive substances from reaching the underlying wire.
- The selection of corrosion-resistant wire materials helps maintain system efficiency and reduces the need for frequent maintenance, ensuring a reliable and long-lasting electromagnetic induction system.
High-Speed Rotational Systems
High-speed rotational systems play a crucial role in the generation of electricity through the utilization of rotational movement to induce electromotive force (EMF). When a magnetic field rapidly rotates around a coil, it generates an alternating current (AC) voltage. The magnitude of the induced EMF is influenced by the speed at which the magnetic field changes and the strength of the magnetic field itself.
In high-speed rotational systems, the magnetic field is typically created by a permanent magnet. As the magnet rotates, the changing magnetic field induces EMF in the nearby coil. This induced EMF opposes the change in magnetic field, in accordance with Faraday’s law of electromagnetic induction. The strength of the induced EMF can be increased by increasing the speed of rotation or by increasing the magnetic field strength.
The number of turns of wire in the coil also affects the magnitude of the induced emf. More turns of wire result in a higher emf, as the magnetic field lines pass through a greater number of wire loops, inducing more voltage. The work done by the induced emf can be harnessed to power various electrical devices.
Enhanced Magnetic Flux Density
Enhanced magnetic flux density can be achieved through various methods and materials. By increasing the number of turns in a coil, the magnetic field strength can be intensified, leading to a higher flux density.
Additionally, using a ferromagnetic core within the coil can further enhance the magnetic field and increase the flux density. Another method to enhance the magnetic flux density is by increasing the current flowing through the coil. This increase in current results in a stronger magnetic field and consequently, a higher flux density.
Furthermore, employing high-permeability materials can help concentrate the magnetic field and enhance the flux density. These materials have the ability to attract and hold magnetic fields, allowing for a more efficient induction process and an increased flux density.
To optimize the design for enhanced magnetic flux density, you can utilize advanced electromagnetic simulation techniques. These techniques provide valuable insights into the magnetic field distribution and allow for adjustments to achieve higher flux density.
Advanced Core Materials
To further optimize the design for enhanced magnetic flux density, the use of advanced core materials becomes crucial in electromagnetic induction systems. These materials play a vital role in maximizing the efficiency and performance of such systems.
Advanced core materials, such as amorphous alloys, nanocrystalline alloys, ferrites, and specialty steel and iron alloys, are specifically engineered to exhibit properties that enable them to oppose the motion of the magnetic field and generate electricity.
In electromagnetic induction, the relationship between electricity and magnetic fields is harnessed. When a magnetic field changes near a conductor, it induces a current in the first conductor. This current flows in the opposite direction to the changing magnetic field, creating an electromotive force (EMF). This EMF generates AC output, which is vital for various applications.
Advanced core materials are designed to maximize the magnetic flux density by ensuring that the magnetic field lines are perpendicular to the magnetic core. This arrangement allows for efficient transfer of energy between the magnetic field and the conductor, resulting in high-performance electromagnetic induction systems.
Furthermore, these materials are engineered to minimize losses and withstand high temperatures and mechanical stresses. They exhibit high permeability, low coercivity, and minimal eddy current losses, ensuring efficient energy transfer and minimizing energy dissipation.
Research in advanced core materials is focused on developing new compositions and processing techniques to meet the increasing demands of modern power generation and distribution systems. The goal is to enhance the efficiency, reliability, and sustainability of electromagnetic induction systems.
Increased Number of Turns
Increasing the number of turns in electromagnetic induction systems can significantly enhance the efficiency and performance of electric generation. By increasing the number of turns in a coil, you can increase the strength of the magnetic field produced when a bar magnet is moved towards the coil.
This is due to Faraday’s Law, which states that the induced electromotive force (EMF) produced is directly proportional to the rate of change of magnetic flux through the coil. Here are four key benefits of increasing the number of turns in electromagnetic induction systems:
- Increased Magnetic Field Strength: With more turns in the coil, the magnetic field generated becomes stronger, resulting in a higher induced EMF. This leads to improved efficiency in electric generation.
- Enhanced Power Output: The increased magnetic field strength translates to a higher power output, allowing for the generation of more electricity.
- Extended Induction Period: More turns in the coil increase the length of time the magnet needs to move to induce the same EMF. This allows for a longer period of time to generate electricity.
- Improved Coil Sensitivity: Multiple coils with an increased number of turns can detect smaller changes in the magnetic field, making the system more sensitive to variations in the magnet’s movement.
Improved Magnetic Shielding
Improved Magnetic Shielding can significantly enhance the effectiveness of electromagnetic induction systems by utilizing high-permeability materials and employing proper design techniques to block unwanted magnetic fields.
When a wire passes through a magnetic field, a back electromotive force (emf) is induced, which opposes the change in magnetic flux. This back emf is caused by the magnetic force acting on the moving charges within the wire.
By reducing the strength of the external magnetic field, the induced back emf can be minimized, allowing for a more efficient transfer of electrical energy.
Proper magnetic shielding can also help maintain the integrity of the output voltage in electromagnetic induction systems. According to the law of conservation of energy, the electrical energy generated by the system is directly proportional to the rate of change of the magnetic field. By shielding the system from external magnetic fields, the induced magnetic field within the system can be more accurately controlled, resulting in a more stable output voltage.
To achieve effective magnetic shielding, you can use high-permeability materials such as mu-metal or ferrite. These materials have the ability to absorb and redirect magnetic fields, preventing them from interfering with the induction process.
Increasing the thickness and coverage of the shielding material can further enhance its effectiveness in blocking unwanted magnetic fields. Additionally, employing proper design techniques, such as magnetic shielding enclosures or cages, can provide comprehensive protection against electromagnetic interference.
Minimization of Eddy Current Losses
How can you minimize eddy current losses in electromagnetic induction systems? Minimization of eddy current losses is crucial in order to optimize the efficiency of electromagnetic induction systems and enhance the conservation of energy. To achieve this, you can employ the following methods:
- Use materials with high electrical resistivity: By utilizing materials that have high resistivity, you can reduce the flow of induced currents within the coil. This helps to minimize the eddy current losses that occur due to the resistance of the conducting material.
- Reduce the thickness of conducting materials: By decreasing the thickness of the conducting materials, you can limit the area affected by changing magnetic fields. This, in turn, reduces the magnitude of the induced EMF and subsequently minimizes the eddy current losses.
- Implement laminated cores: Laminated cores are a common technique to minimize eddy current losses. By isolating the conducting materials with insulating layers, the formation of large, circulating eddy currents is reduced. This effectively mitigates the losses associated with these currents.
- Utilize materials with high permeability: Magnetic materials with high permeability can confine and channel the magnetic flux within the coil. This helps to minimize the impact of eddy current losses by directing the magnetic field and reducing the formation of circulating currents.
Conclusion
By implementing the methods mentioned, such as magnetic field manipulation, coil design optimization, and efficient wire materials, we can greatly improve the efficiency and effectiveness of electric generation through Faraday induction.
Remember, as the saying goes, ‘A stitch in time saves nine.’ Therefore, investing in these advancements won’t only enhance our ability to generate electricity but also contribute to a more sustainable and reliable energy future.
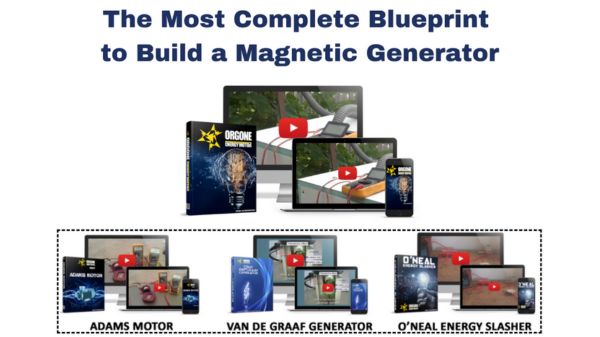